Models of eel cells suggest electrifying possibilities Thursday, 09 October 2008 Researchers at Yale University have created a blueprint for artificial cells that are more powerful and efficient than the natural cells they mimic and could one day be used to power tiny medical implants. The scientists began with the question of whether an artificial version of the electrocyte – the energy-generating cells in electric eels – could be designed as a potential power source. "The electric eel is very efficient at generating electricity," said Jian Xu, a postdoctoral associate in Yale's Department of Chemical Engineering. "It can generate more electricity than a lot of electrical devices." Xu came up with the first blueprint that shows how the electrocyte's different ion channels work together to produce the fish's electricity while he was a graduate student under former Yale assistant professor of mechanical engineering David LaVan, now at the National Institute of Standards and Technology. But the scientists didn't stop there. "We're still trying to understand how the mechanisms in these cells work," said LaVan. "But we asked ourselves: 'Do we know enough to sit down and start thinking about how to build these things’ — and to optimize that design? Nobody had really done that before." Using the new blueprint as a guide, LaVan and Xu set about designing an artificial cell that could replicate the electrocyte's energy production. "We wanted to see if nature had already optimized the power output and energy conversion efficiency of this cell," said Xu. "And we found that an artificial cell could actually outperform a natural cell, which was a very surprising result." The artificial cell LaVan and Xu modelled is capable of producing 28 percent more electricity than the eel's own electrocyte, with 31 percent more efficiency in converting the cell's chemical energy – derived from the eel's food – into electricity. Electric eels channel the output of thousands of specialized cells called electrocytes to generate electric potentials of up to 600 volts, according to biologists. The mechanism is similar to nerve cells. The arrival of a chemical signal triggers the opening of highly selective channels in a cell membrane causing sodium ions to flow in and potassium ions to flow out. The ion swap increases the voltage across the membrane, which causes even more channels to open. Past a certain point the process becomes self-perpetuating, resulting in an electric pulse travelling through the cell. The channels then close and alternate paths open to “pump” the ions back to their initial concentrations during a “resting” state.
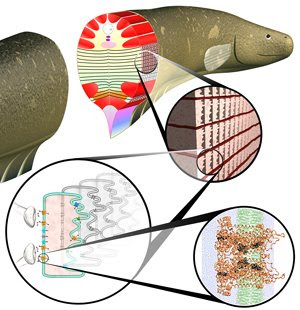
In all, according LaVan, there are at least seven different types of channels, each with several possible variables to tweak, such as their density in the membrane. Nerve cells, which move information rather than energy, can fire rapidly but with relatively little power. Electrocytes have a slower cycle, but deliver more power for longer periods. LaVan and Xu developed a complex numerical model to represent the conversion of ion concentrations to electrical impulses and tested it against previously published data on electrocytes and nerve cells to verify its accuracy. Then they considered how to optimize the system to maximize power output by changing the overall mix of channel types. Their calculations show that substantial improvements are possible. One design for an artificial cell generates more than 40 percent more energy in a single pulse than a natural electrocyte. Another would produce peak power outputs over 28 percent higher. In principle, say the authors, stacked layers of artificial cells in a cube slightly over 4 mm on a side are capable of producing continuous power output of about 300 microwatts to drive small implant devices. The individual components of such artificial cells — including a pair of artificial membranes separated by an insulated partition and ion channels that could be created by engineering proteins — already have been demonstrated by other researchers. Like the natural counterpart, the cell’s energy source would be adenosine triphosphate (ATP), synthesized from the body’s sugars and fats using tailored bacteria or mitochondria. While eels use thousands of electrocytes to produce charges of up to 600 volts, LaVan and Xu’s calculations show it would be possible to create a smaller "bio-battery" using several dozen artificial cells. The tiny bio-batteries would only need to be about 4 mm thick to produce the small voltages needed to power tiny electrical devices such as retinal implants or other prostheses. Although the engineers came up with a design, it will still be some time before the artificial cells are actually built. For one thing, they still need a power source before they could start producing electricity. LaVan speculates the cells could be powered in a way similar to their natural counterparts. It is possible, he said, that bacteria could be employed to recycle ATP – responsible for transferring energy within the cell – using glucose, a common source of chemical energy derived from food. With an energy source in place, the artificial cells could one day power medical implants and would provide a big advantage over battery-operated devices. "If it breaks, there are no toxins released into your system," said Xu. "It would be just like any other cell in your body." Reference: Designing artificial cells to harness the biological ion concentration gradient J. Xu and D.A. LaVan. Nature Nanotechnology advanced online publication 10.1038/nnano.2008.274 ......... ZenMaster
For more on stem cells and cloning, go to CellNEWS at http://cellnews-blog.blogspot.com/ and http://www.geocities.com/giantfideli/index.html
No comments:
Post a Comment